
In the 80’s and 90’s, the Partnership for a Drug-Free America released several public service announcement commercials, which aimed to inform the public about the dangers of drugs of abuse. The commercials, which have made a lasting impact on society and pop culture, featured a shot sequence and narration of your brain (an uncracked egg), drugs (a hot frying pan), and your brain on drugs (a cracked and frying egg).
Because substance abuse either directly or indirectly affects virtually every person in our society, the National Institutes of Health (NIH) puts a lot of funding toward understanding the neurobiological mechanisms of drug abuse. This has led to some really interesting discoveries about the mechanisms by which drugs of abuse can ‘hijack’ our brains. Today, I’m going to focus on a big finding about drugs of abuse that interestingly deals with something too tiny for most microscopes to see: DNA.
Before diving into the mechanisms of drug abuse, we need to first talk a little about DNA. There’s a lot of confusion about how DNA works, possibly in part due to the way it’s been represented in pop culture. Hollywood likes to use DNA as an explanation for a lot more than it’s capable of (I’m thinking Spiderman, Hulk, X-Men, etc…). In reality, DNA is an incredibly long molecule containing a “code” of sorts, which consists of 4 distinct chemicals with different structures, denoted as A, C, G and T. When reading the code, it looks something like this: “aattagaccggataataca,” and has a reverse, complementary string going in the opposite direction. When inside a cell, DNA is wound up into a highly condensed structure called chromatin, which is similar to a ball of yarn.

DNA is the blueprint for proteins, which are the little molecular machines that do most of the work on a cellular level in the body. The process of making a protein from DNA, sometimes known as the central dogma of molecular biology, involves making a temporary copy of the DNA blueprint (called messenger RNA, or mRNA) and then taking that copy to a molecular factory called a ribosome, where the protein is made. You can think of this process like a car-making operation: somebody designs a car and has master instructions for how to design and assemble all the parts. These instructions are kept in a master library of car designs, and temporary instructions are copied on site (in our analogy, this has to be done by hand because computers haven’t been invented yet). Someone then arrives with the temporary copy, which represents mRNA, to the factory, and parts are assembled to make a fully-functional car that can cruise the streets. Depending on the master instructions and the design, parts of the car can be altered, removed, added, or whole different models can be created. This process of copying a master code, delivering it to a factory, and then turning the temporary code into a functional protein is called translation and transcription. Here is an incredibly well-drawn cartoon to illustrate:

Transcription and translation are tightly-controlled processes. So, if you send more mRNA to the ribosome factories, then more proteins will be created (called upregulation); and if you send less mRNA to ribosome, then less proteins will be created (called downregulation). Or, if you send improperly-coded mRNA to the factory or the original DNA template code is faulty, it will result in proteins that don’t work quite right, like a car without an engine or just 3 wheels. What does it mean for an organism when this process gets out of whack? Problems with production in proteins can have mild to drastic consequences, including improper development or death.
Drugs of abuse; especially nicotine, cocaine, heroin, methamphetamine, and alcohol; all have a host of genes that they upregulate and downregulate. Because of the distinct molecular shapes and functions of different abusable substances, they all affect the release of dopamine, a neurotransmitter involved in feeling pleasure, reward, and mood, in slightly different ways. However, most (if not all) drugs of abuse induce and up-regulation of a protein called delta fos B(ΔFosB).
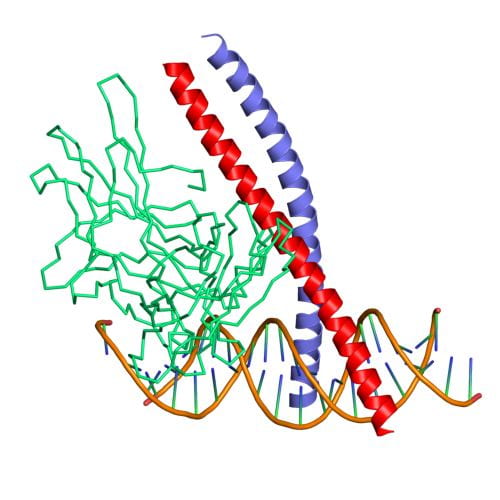
ΔFosB is a transcription factor, meaning that it assists in the process of copying the DNA template onto temporary mRNA molecules and increasing or decreasing the production of various proteins. The picture above shows it and some other transcription factors stuck to a DNA molecule. ΔFosB has actually been shown to play an important role in many basic biological process that are related to reward and/or motivation, such as food or sex. However, after repeated exposure to drugs of abuse, ΔFosB accumulates in brain regions important for motivation and reward. As it piles up in these brain regions, it affects up-regulation and down-regulation of other genes. It is thought to affect how neurons communicate by increasing amounts of the gene GluR2, which can decrease a neuron’s electrical excitability. Additionally, ΔFosB can upregulate a natural opioid called dynorphin, which is linked to depressed mood and might contribute to the long-term negative affect (i.e. depression) that is often observed after drug abstinence.
Substance abuse affects everyone in society; if not directly, then indirectly through its societal costs. It often accompanies mental illness, broken families, homelessness, criminal activity, and systemic oppression. Furthermore, it plays a role in both national and international politics. Thus, it is important that we keep in mind how individual occurrences of drug abuse can have lasting societal and worldwide impacts. While we’re tackling the world-scale issues around drug abuse, I hope we can keep in mind the microscopic occurrences that have tremendous consequences.
Edited by Kat Munley and Katherine VanDenBurgh
Leave a Reply