This post was written by Jorge Morales.
In 1916, Einstein predicted there are ripples in the fabric of space that travel through the universe at the speed of light. Today we know those ripples as gravitational waves. A century after Einstein’s prediction, science gave Einstein one of his biggest victories: the detection of gravitational waves. The detection came from gravitational waves emitted by black holes, which are the densest astrophysical bodies known in the universe, so dense that even light can’t escape their gravitational pull. Two black holes with a total mass of 65 solar masses (or 22 million Earth masses) danced around each other, until they collided to form a new black hole of 62 solar masses. The mass of approximately three solar masses was converted into the enormous energy that these gravitational waves carried. These waves traveled through space for more than a billion years, until the Laser Interferometer Gravitational-wave Observatory (LIGO) was able to catch them on September 14, 2015.
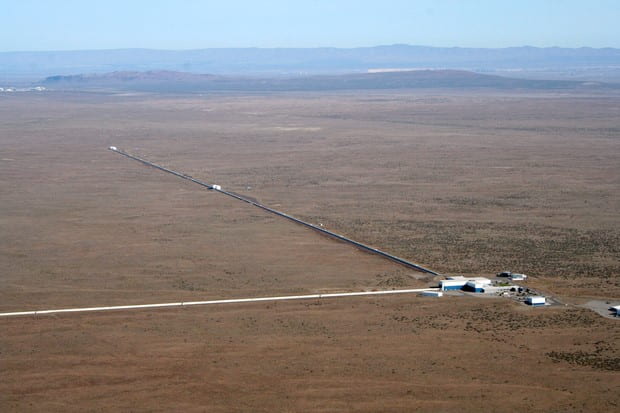
This state-of-the-art instrument detected these waves by observing changes in distance about 10,000 times smaller than the diameter of a proton. Just so you have an idea of how small that is: the diameter of the proton is to the height of a human, as the thickness of a sheet of paper is to the distance to the Sun. This extraordinary detection inaugurated the gravitational wave astronomy era in science. Since then, the technology of LIGO and similar detectors have improved in such a way that observing black hole collisions is the bread-and-butter of gravitational wave astronomers. Furthermore, LIGO and other gravitational wave detectors even observed the collision of two neutron stars, which was unlikely to be detected. However, despite all of this progress, instruments haven’t been able to hear the elegant, almost constant, and well-defined whistle of a continuous gravitational wave.
The cataclysmic collision of black holes and neutron stars produce a blast of different frequencies, that last hundreds of seconds at most, and can be detected by LIGO and other similar instruments. Unlike this complicated burst of gravitational waves, continuous gravitational waves are associated with a steady frequency that can last much longer. For instance, you might visualize the burst of gravitational waves as the sound of two cars crashing, while a continuous gravitational wave is like the sound of a sustained piano key. Continuous gravitational waves are hard to detect because they are weak, but their detection would be fruitful. First, since a continuous gravitational wave has a simpler mathematical description than a burst of gravitational waves, it provides a simpler framework to compare theories (mathematical models of how nature behaves) to experiments (observations of how nature works). That means continuous gravitational waves could be used to test the limits of Einstein’s theory of gravity and the predictions of theories that go beyond Einstein (for example, the Standard Model Extension theory developed by IU professor Alan Kostelecky). Another landmark that could be achieved with the detection of continuous gravitational waves is the study of the astrophysical bodies that emit them. Studies have suggested that neutron stars could have various mechanisms in which they emit continuous gravitational waves. For example, IU professor Charles Horowitz has been studying the outer solid layer of neutron stars, better known as the crust. On numerous occasions he has concluded that tiny bumps on the crust, approximately 1 cm high (better known as mountains), of rapidly spinning neutron stars, that are approximately 3,000 light years away, can lead to the emission of detectable continuous gravitational waves. If we detect waves from neutron star mountains, it can improve what we know about the crust and the structure of neutron stars.
Because of the high rewards of the detection of continuous gravitational waves, numerous groups around the world have been searching for them. Although these searches have not yet been successful, improvements to current gravitational wave detectors and plans to construct more sensitive detectors will increase our odds of catching them. For example, the Einstein Telescope, which is expected to start operations in 2035, will be an underground gravitational wave detector, 10 times more sensitive than current detectors. With this telescope, we’ll be able to catch gravitational waves that are 10 times weaker than those that current detectors can observe, which in turn gives us much higher chances of detecting the weak continuous gravitational waves.
Physicists are intrigued by the realistic possibility of observing continuous gravitational waves. Can it be that these waves show the pitfalls of Einstein’s theory of gravity? Would these waves point us to a novel fundamental theory of gravity? Will we be able to observe these signals from the crust of neutron stars? What information about the crust would they encode? To answer these and other questions, we are on the hunt for continuous gravitational waves.
Acknowledgements:
Thanks to Prof. Charles J. Horowitz for helping me in editing this article.
Edited by Chloe Holden and Taylor Woodward
Leave a Reply